Quantum of cytotoxicity - in dots and dashes
- Mythili Tummalapalli
- Oct 27, 2016
- 3 min read
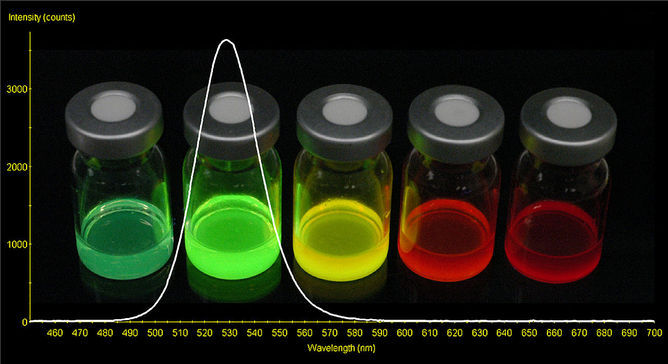
Image copyright: NASA
Quantum dots (QDs) have been around since the late 1960s, and over the past few decades, they have opened up new vistas of application, including fuel cells, LED displays, quantum computing, and in vivo imaging. The unique photoluminiscent properties of QDs can provide a huge fillip to clinical imaging; especially in the field of theranostics, which combines diagnostics and therapy. Much attention has been paid to cadmium based QDs (including CdSe, CdS, and CdTe) as in vivo imaging agents for cancerous tumors. However, the cytotoxic effect of these nanocrystal semiconductors poses a large (band) gap (sic) that has to be surmounted for them to be accepted widely in biomedical applications.
The cytotoxic effects of Cd based QDs have been well explored and several mechanisms have been proposed to explain the genotoxic, epigenetic and metalloestrogenic effects of Cd based QDs. A recent study using chorioallantoic membrane separated from chicken embryos demonstrated the embryotoxic and cytotoxic responses induced on exposure to QDs, unless used at extremely low concentrations [1]. QD nanoparticles smaller than 80-100 nm can escape phagocytosis, and are capable of penetrating and accumulating in fenestrated tissues such as kidney and liver. The accumulated deposits subsist over long periods of time and can cause epigenetic effects. In one particular study, it was observed that the QDs accumulated in the liver are capable of crossing the placental barrier in mice [2]. Secondly, in a richly oxidative environment, such as the tumor microenvironment, Cd2+ ions are released from the QD surface and bind to the sulfhydryl groups of mitochondrial proteins, leading to necrosis [3]. The effect is exacerbated if the surface of the nanocrystals is irregular. Such factors cause justified apprehension and thus far, only one QD based product has been cleared for clinical trials.
Nevertheless, it is reasonable to think that we now have a good understanding of the possible cytotoxic mechanisms and can take necessary steps to proactively mitigate or even inhibit adverse effects. A multitude of factors can be considered, including the QD synthesis conditions, QD size, surface modification, and encapsulation (polymeric or liposomal). Bhatia’s group at UCSD comprehensively proved that Cd based QDs synthesized under strictly inert conditions did not inhibit hepatocyte cell proliferation [4]. Polyethylene glycol (PEG) is widely used as a capping agent on QDs to increase their circulation time in the bloodstream as well as to reduce the cytotoxicity. However, PEG cannot stop the diffusion of released Cd2+ ions; therefore, maybe it is time for us to develop materials that can actively immobilize Cd2+ within their matrix. For example, biopolymers that can chelate cadmium are used for heavy metal separation from waste water. Why not employ or develop materials that are functionally similar? Protein engineering is now a by-word in biomedical research. Can we design and synthesize amino acid sequences that can render Cd2+ inert in physiological conditions, à la metallothionene? Such ligands may be immobilized either on native Cd based QDs or the QD capping agent via simple coupling reactions. At the same time, capped Cd based QDs with faster detection rates, and renal elimination times may be developed. It’s time to think cross-disciplinary if the potential of QDs is to be used for theranostics!
References
Blažkováa, I. et al. Application of quantum dots into chicken embryos. Journal of Metallomics and Nanotechnologies 2014; 3: 26-28.
Chu, M. et al. Transfer of quantum dots from pregnant mice to pups across the placental barrier. Small 2010; 6: 670-678.
Derfus, A. M. et al. Probing the cytotoxicity of semiconductor quantum dots. Nano Letters 2004; 4: 11-18.
Winnik, F. M. and Maysinger, D. Quantum dot cytotoxicity and ways to reduce it. Accounts of Chemical Research. 2013; 46: 672-680.
© Mythili Tummalapalli, 2016.
Comments